In search of the fundamental scientific laws that predict biological prosperity from physics, biology, sociology, economics, neuroscience and intelligence.
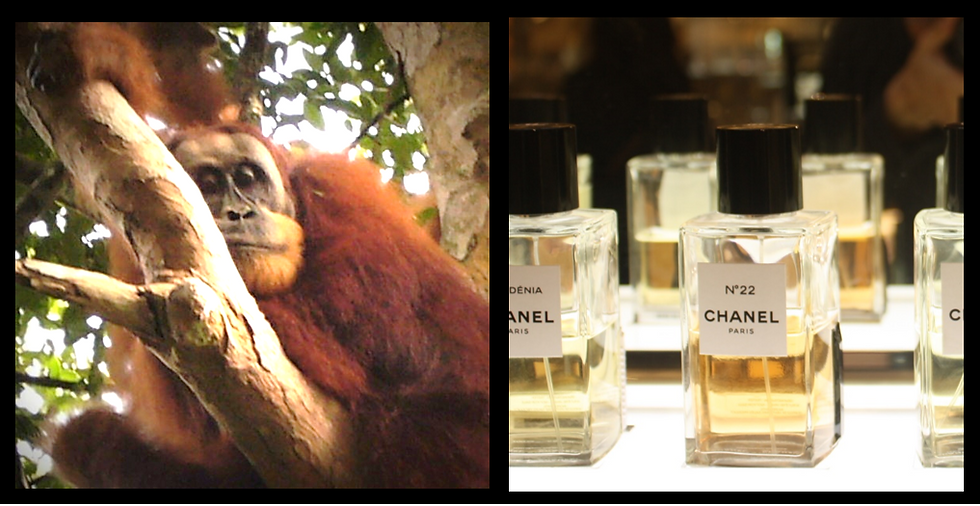
Two images that you can still find in 2019 - an orangutan in natural habit on Sumatra, and luxury brand perfume in the central floor of Galeries Lafeyette in Paris. How likely will similar images like these survive into the next millennial given what we know about the science of climate change, urbanization, economics and human behavior?
This article is an exploration of ideas for ways to respond to this question in continuation of the ideas presented in a recently published book by Geoffrey West : Scale The universal laws of growth and death in organisms, cities and companies.
The defining narrative for our times will be how we as humans - a biological, social, innovative and moral species respond to the threat of anthropogenic global warming tactfully and responsibly in the context of an urbanizing civilization. Borrowing a useful hueritsic from engineering :
A well defined problem statement is an 80% solved problem
The IPCC report in October 2018 has laid out the key details of the problem statement of global warming, including "safe" level of warming definition 1.5C, a budget of remaining GHG emissions and a timeline to net zero 2050. The roadmap to net zero can be summarized as a combined effort of implementing win-win projects that achieve progress in the road to zero, and are also inherently financially profitable, together with building the political will to execute projects that do not have a clear connection to short term financial motives. Electrifying machinery, powering grids with renewable electricity, improving energy efficiency alone unfortunately may not be enough to meet the tight drawdown timeline required by the IPCC report without considering reforestation, carbon capture and restrictions on further oil and gas well exploration. Rephrasing the conclusion from a recent report by ExxonMobil on climate change
The forecasted global energy demand is unlikely to be fully met by renewables and the natural draw-down of existing production wells given trends of population, urbanization growth and our understanding of the carbon intensity of GDP
How much do we understand about the fundamentals of cities, GDP, social behavior, innovation and individual humans and how these dynamics relate to the constraints of the reality check that we operate in a closed system on one irreplaceable planet with finite resources and biocapacity?
Hoping for a pricing signal to naturally emerge to internalize this externality risk may be wishful thinking for the inconvenient truth of global warming, given the current track record. Evidence for this pessimistic assumption can be found from the observed lack of change in trajectory of GHG emissions at global level and by theory given what we know about the timescales and geographic reach of the manifestation of climate change, and from Prospect Theory that humans decision under risk is not always rational.
If we consider a systematic design based approach to drawdown the energy intensity of GDP, exactly how would we go about it? How to make the best decisions about which socioeconmic activites - consumption, industries, and skills to promote, and which to restrict? What are the risk?
To answer this design challenge may require both a revisting of values and concepts of prosperity and a deeper understanding of the scientific laws of physics, biology, sociology, economics, neuroscience and intelligence.
Redefining prosperity
Consider variations in concepts of prosperity from a biological, social and financial perspective. A biological basis for prosperity could be the fitness of a species on a planet of finite resources. A social definition of prosperity may incorporate concepts of power, influence, contribution, altruism and empathy.
Financial prosperity is a dominant perspective and associated with terms such as wealth and measures such as real GDP per capita, earnings, labor productivity and real wages. Part of the goals of economics as a field is to interpret meaning for financial profit and fundamentally a pricing signal is inherently a socially constructed measure and depends on the collective wisdom of individuals to incorporate the physical reality of their environment and translate that experience into a hedonic/utility pricing dynamic. Pricing dynamics are not guaranteed to reflect biological or social prosperity measures. It may be reasonable to assume that currently the socially defined prices of goods and services are not reflecting the existential risk of climate change and GHG emissions, so that an endeavor purely driven in the name of financial prosperity, without accounting for hard constraints of the natural world, may one day face an unpleasant and brutal suprise reality check. If history is a predictor of the future, there are many examples in the past of civilizations that have faced major losses because of failing to anticipate existential risks that were not captured in their parochial business as usual decision making paradigm.
Given a biologically and socially meaningful definition of prosperity, how then with mathematical precision and certainty to guide tactical decisions for the next 30 years as a policy maker, shareholder, educator, innovator, manager, employee, consumer, friend, family member and a person ?
Thermodynamics and the Navier Stokes model of cities
The Navier Stokes model is used to predict fluid dynamics phenomenon and combined what was already known from Newton's laws of physics and other emerging disciplines into a single partial differential equation. It was developed at a time before it could be practically solved and applied to real world problems in its general form, and for a long time its practical implementations in fluid dynamics engineering problems were simplified approximations of the general equation and define many of the common engineering equations that helped to develop the technology that runs cities. The model is frequently applied in the field of Chemical Engineering - a field which has provided the technological means for 20th century urbanization by fueling cities with a cheap and abundant source of energy (petrochemical industry) and food (Haber Bosch). To quote my professor from university :
One of the unique features of Chemical Engineering field is that addresses design at a wide range of scales compared to other Engineering displines.
- Ronald Rousseau
Navier stokes is one example of the power of a scalable model. Techniques for scaling frequently employ dimensionless parameters such as Prandlt number and Reynolds number that characterize phenomenon on an atomic level to predict emergent system level behavior. Developing a model that scales well is a recurring challenge in research. Certain phenomenon that are dominant at micron levels dissapear into negligence at larger scales and visa-versa. Also in the development of models simplifying assumptions are necessary, and as a consequence of the simplification the scale of models frequently is bounded to these assumption limits. For these reasons there is a limited amount of scientific models that can be confidently scaled to atomic and planetary levels. Scalability requires a solid theoretical foundation, and a natural starting point for a scalable model of cities that predicts energy demand is Thermodynamics.
If your theory is found to be against the second law of Thermodynamics, I can give you no hope; there is nothing for it but to collapse in deepest humiliation
- Albert Einstein
Thermodynamics and Chemical Engineering trace their origins to the work of Josiah Willard Gibbs. Thermodynamics is summarized into four laws (0,1,2,3). The second law defines entropy and that for any closed system entroy is always increasing with the passage of time. Fundamental to a thermodynamics design approach is the concept of equilibrium, that although the detailed mechanisms of how a system reaches a particular state may not be well understood or predicted, knowing the direction and final state may be all that is needed practically. Another concept of equilibrium for systems is that while there may be wide, complex diversity of features of individuals, their aggregate properties may conform to simple predictable patterns.
Equilibrium and thermodynamic based models have been extended and applied in range of fields such as biology and economics. The general technique for developing a thermodynamic based model is to define the relevant forces in the system, the range of possible states and apply the theory to predict the nature of the equilibrium state where the forces are balanced and small disturbances return back to the locally optimal equilibrium.
In the book "Scale" Geoffrey West presents universal scaling emperical observations for the metabolism of animals vs their size, and explains these scaling trends from the fundamentals of phenomenon at the smallest scale - the terminal units and the fractal geometry of their network structure. For biological systems the terminal unit is the interface between capilaries and cells. Later in the book he applies a similar approach to derive scaling laws for cities also based on their fractal network geometry and the nature of the terminal units - households. The results of the analysis present evidence that emergent features of cities - GDP, crime, energy consumption, land use can all be predicted by applying these scaling laws and knowing the city's population size. The suprising result that all of the features trace to a common scaling factor of either 1.15 (+0.15) for socioeconomic measures, or 0.85 (-0.15) for metabolic infrastructure measures suggests a common dynamic is occuring on multiple scales and related to characteristics of social and infrastructure networks. To verify these claims I tested this theory on a public data set of global cities using the city population size and area. The results of this adhoc exercise was that urban area scales sublinearly with population by a factor 0.78 and this slope is universal in all global cities. Another clear observation was that compared to the rest of the world, United States, Australia and Canada are on different curves, while sharing the same slope. In other words, at all scales US cities occupy more land area than other cities in the world of the same population size. The opposite is true India and Bangladesh, which have unusually smaller area relative to their population size although their difference from the global average is smaller compared to the US. Aside from these countries, all other countries have a similar predictable size-land area relationship.
A take-away conclusion from the book is that the majority of what is captured as GDP growth can be explained in terms of urbanization as the physical and social development of cities. Cities develop in a pattern that preferentially economizes fixed costs of infrastructure and supply of basic operating costs such as energy and food, and maximizes the opportunity for social contact and exchange. GDP can be interpreted both in terms of real wages and in terms of the velocity of money, both of which are related to the volume of financial transactions of residents in the city - both within the city and with external connections outside the city. GDP metrics at national level can be confusing, but once the urbanization patterns are accounted for GDP differences become more predictable. Another insight is that cities have an immortal quality and persist for centuries with a continous uninterrupted metabolism and physical presence. The book cites London, Hiroshima and Nagasaki as examples that were decimated by bombing, but yet resumed and are again vibrant and metabolizing.
The book stops however at defining a predictive model for the energy intensity of social exchange Although a model that predicts higher rate of social exchange does not guarantee an increase in GDP or real wages, following from the conclusions from Scale this may be a useful starting point.
The book also stops at describing an objective valuation metric to discriminate the different ranges of good and services produced through social exchange. For example in the case of an MRI machine in a hospital and perfume in a shopping mall. Both have a quantifiable valuation in socially defined financial metrics, and both have a quantifiable energy intensity. Is there a measure of biological prosperity of the city that can quantify the marginal opportunity cost of species fitness between these two products? Can innovative capacity, learning and problem solving potential be objectively measured at the scale of individuals and cities and can its energy intensity be quantified?
A Navier Stokes model of cities incorporating the predictable, scalable dynamics in physics, biology, economics, sociology, neuroscience and intelligence may be an approach to guiding the answers to these questions. While this goal may sound ambitious, the examples of Gibbs and Geoffrey provide a clue that it may not be beyond the reach of directed research with the tools of computing and the body of existing knowledge in these respective fields.
Comments